Neutron stars are some of the densest objects in the Universe. The material inside is squeezed so hard that scientists don’t yet know what shape it takes. The core of a neutron star may consist of a thick soup of quarks, or contain exotic particles that cannot survive anywhere else in the Universe. Credit: ICE-CSIC/D. Futselaar/Marino et al., edited
Recent observations from ESA’s XMM-Newton and NASAChandra has discovered three new extremely cool neutron stars, challenging current models by showing that they are cooling much faster than expected.
This finding has important implications, suggesting that only a few of the many proposed neutron star models are viable and point to a possible advance in linking the theories of general relativity and quantum mechanics through astrophysical observations.
Discovery of unusual cool neutron stars
ESA’s XMM-Newton spacecraft and NASA’s Chandra spacecraft have discovered three young neutron stars that are extremely cool for their age. By comparing their properties with various models of neutron stars, the scientists conclude that the low temperatures of random balls disqualify about 75% of known models. This is a major step towards discovering the ‘equation of state’ of a neutron star that rules them all, with important implications for the fundamental laws of the Universe.
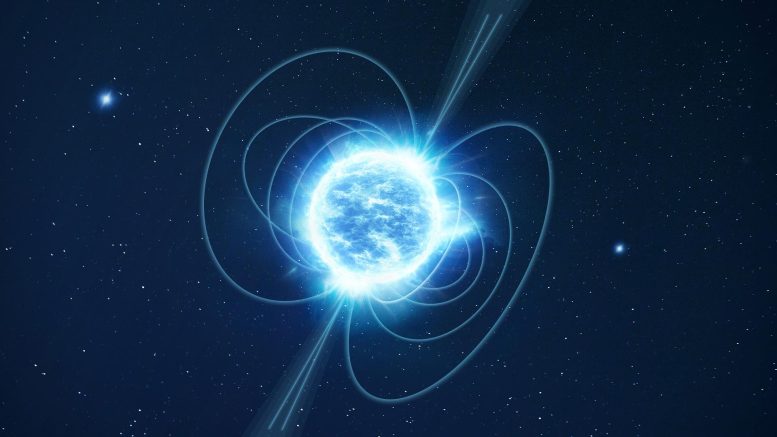
Apart from black holes, neutron stars are among the most puzzling objects in the Universe. A neutron star forms in the final moments of the life of a very large star (more than eight times the mass of our Sun), when the nuclear fuel in its core eventually runs out. In a sudden and violent end, the outer layers of the star are ejected with monstrous energy in a supernova explosion, leaving behind spectacular clouds of interstellar material rich in dust and heavy metals. At the center of the cloud (nebula), the dense stellar core contracts further to form a neutron star. A black hole can also form when the remnant mass of the core is greater than about three solar masses. Credit: ESA
Extreme densities and unknown states of matter
After stellar-mass black holes, neutron stars are the most dense objects in the Universe. Each neutron star is the compressed core of a giant star, left behind after the star exploded in a supernova. After running out of fuel, the star’s core explodes under the force of gravity, while its outer layers are blasted outward into space.
The matter at the center of a neutron star is squeezed so tightly that scientists still don’t know what shape it takes. Neutron stars get their name from the fact that under this tremendous pressure, even atoms collapse: electrons fuse with atomic nuclei, turning protons into neutrons. But it can get even weirder, as extreme heat and pressure can stabilize more exotic particles that don’t survive anywhere else, or perhaps fuse the particles together into a swirling soup of their constituent quarks.
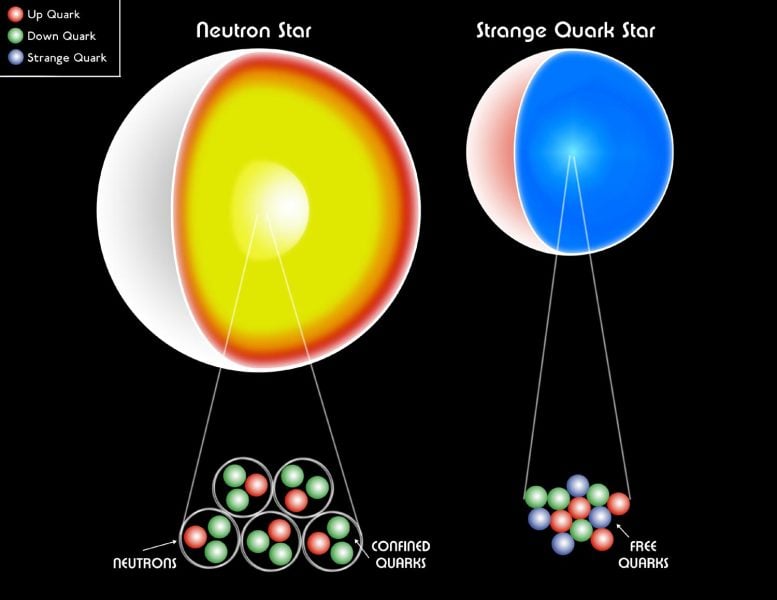
In a neutron star (left), the quarks that make up the neutrons are confined within the neutrons. In a quark star (right), the quarks are free, so they take up less space and the diameter of the star is smaller. Credit: NASA/CXC/M.Weiss
What happens inside a neutron star is described by the so-called ‘equation of state’, a theoretical model that describes what physical processes can take place inside a neutron star. The problem is that scientists don’t yet know which of the hundreds of possible equation of state models is correct. While the behavior of individual neutron stars may depend on properties such as their mass or how fast they spin, all neutron stars must obey the same equation of state.
Implications of Observations of Neutron Star Cooling
Digging into data from ESA’s XMM-Newton missions and NASA’s Chandra missions, scientists discovered three extremely young and cool neutron stars that are 10-100 times cooler than their peers of the same age . By comparing their properties with the cooling rates predicted by various models, the researchers conclude that the existence of these three random fronts rules out most of the proposed equations of state.
“The young age and cold surface temperature of these three neutron stars can only be explained by invoking a rapid cooling mechanism. Since extended cooling can only be activated by certain equations of state, this allows us to rule out a significant part of possible models,” explains astrophysicist Nanda Rea, whose research group at the Institute of Space Sciences (ICE-CSIC ) and the Space Institute. Studies of Catalonia (IEEC) led the investigation.
Unifying theories through the study of the neutron star
The discovery of the neutron star’s true equation of state also has important implications for the fundamental laws of the Universe. Physicists don’t yet know how to reconcile general relativity (which describes the effects of gravity on large scales) with quantum mechanics (which describes what happens at the particle level). Neutron stars are the best testing ground for this as they have far more density and gravity than anything we can create on Earth.
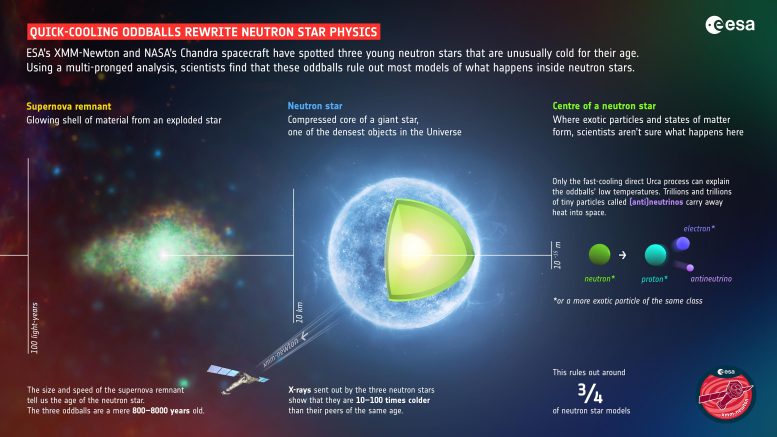
Neutron stars are the compressed cores of giant stars, left over after the star explodes in a supernova. They are so dense that the amount of neutron star material in a sugar cube would weigh as much as all the people on Earth! Credit: ESA
Joining Forces: Four Steps to Discovery
The three strange neutron stars being so cool make them too faint to be seen by most X-ray observatories. “The excellent sensitivity of XMM-Newton and Chandra made it possible not only to detect these neutron stars, but collecting enough light to determine their temperatures and properties,” says Camille Diez, ESA researcher working on the XMM-Newton data.
However, the sensitive measurements were only the first step toward reaching conclusions about what these random balls mean for the neutron star’s equation of state. To this end, Nanda’s research team at ICE-CSIC combined the complementary expertise of Alessio Marino, Clara Dehman and Konstantinos Kovlakas.
Alessio led the way in determining the physical properties of neutron stars. The team could deduce the temperatures of the neutron stars from the X-rays sent off from their surfaces, while the sizes and velocities of the surrounding supernova debris gave a precise indication of their age.
Clara then took the lead in calculating neutron star ‘cooling loops’ for equations of state involving various cooling mechanisms. This involves plotting what each model predicts about how a neutron star’s luminosity—a characteristic directly related to its temperature—changes over time. The shape of these curves depends on several different properties of a neutron star, not all of which can be accurately determined from observations. For this reason, the team calculated cooling curves for a range of possible neutron star masses and magnetic field strengths.
Finally, a statistical analysis led by Konstantinos put it all together. Using machine learning to determine how well the simulated cooling curves match the properties of oddballs, showed that equations of state without a rapid cooling mechanism have zero chance of matching the data.
“Neutron star research crosses many scientific disciplines, ranging from particle physics to gravitational waves. The success of this work shows how fundamental teamwork is to advancing our understanding of the Universe,” concludes Nanda.
Reference: “Constraints on the equation of state of dense matter from young and cold isolated neutron stars” by A. Marino, C. Dehman, K. Kovlakas, N. Rea, JA Pons and D. Viganò, 20 June 2024 , Astronomy of Nature.
DOI: 10.1038/s41550-024-02291-y