Researchers at ETH Zurich have successfully demonstrated the high-vacuum levitation of a silica nanoparticle on a photonic-electric hybrid chip. This important achievement, detailed in their latest study published in Nanotechnology of naturerepresents a major step forward in the field of nanotechnology and opens up new possibilities for future technological applications.
The discovery is the latest in a series of advances in nanotechnologyprompting some leading futurists to predict that developments in biotechnology, artificial intelligence and nanobots will significantly affect the future of humanity in the coming years.
“Vacuum levitation has evolved into a versatile technique… [and] It holds great promise for advancing the study of quantum mechanics in the unexplored macroscopic regime,” the authors of the study write. “However, most current levitation platforms are complex and large.”
“Here we show high-vacuum levitation and motion control of a silica nanoparticle on the surface of a hybrid optical-electrostatic chip.”
The ETH Zurich team’s hybrid chip consists of two layers: an upper photonic layer where the nanoparticle is trapped and detected, and an electrical lower layer with planar electrodes for feedback cooling.
This setup allows accurate detection of nanoparticle motion by analyzing scattered light. This method achieves high signal-to-noise ratios without the need for large, high numerical aperture lenses.
In practical terms, the photonic layer consists of four orthogonally split mode optical fibers. These fibers form standing waves that create multiple trapping sites, effectively canceling dispersion forces and providing strong particle confinement. The bottom layer uses electrodes to cool the reaction, stabilizing particle motion in three dimensions and allowing precise control.
This hybrid photonic-electric platform allows robust lift-off, precise position detection and dynamic control of the nanoparticle in a vacuum without bulky optical devices.
This compact design may make the technology more practical for real-world applications, including portable devices and confined spaces such as cryostats.
The main advantage of this new method of vacuum levitation lies in the integration of optical and electrostatic components on a single chip, allowing high precision and control over the movement of nanoparticles.
While this discovery focuses primarily on microscopic particles, the word “levitation” raises intriguing questions about its implications for larger flight technologies, including advanced propulsion systems.
Microscopic vacuum levitation, such as The levitation of silica nanoparticles demonstrated in this latest study is fundamentally different from the larger-scale levitation that people might associate with science fiction concepts like flying cars or “antigravity.”“ ships.
At the microscopic level, levitation is achieved using precise control of electromagnetic fields and laser cooling techniques within highly controlled environments, usually under vacuum conditions. These methods focus on counteracting the forces acting on small particles, allowing them to float or suspend without physical contact.
In contrast, levitation on a larger scale, such as that envisioned for exotic flying vehicles or spacecraft, would require overcoming the gravitational force acting on much larger masses.
This is likely to involve completely different principles, such as magnetic levitation (maglev), which uses powerful magnets to lift and propel vehicles, or possible future technologies, which are currently theoretical.
The engineering and energy requirements for such large-scale levitation are exponentially more significant, and the environmental conditions are more varied and challenging to control compared to a vacuum-sealed laboratory facility.
After all, microscopic levitation is a well-studied and practical technique with existing technological applications. Large-scale levitation, such as flying cars or “antigravity”.“ technologies, remains theoretical.
Instead of trying to achieve levitation, most experts working on next-generation propulsion systems are focusing on concepts like functional deforming discs and hybrids plasma propulsion systems.
That said, the ability to lift and control nanoparticles under high-vacuum conditions could revolutionize several fields, including quantum computing, materials science, and precision sensing.
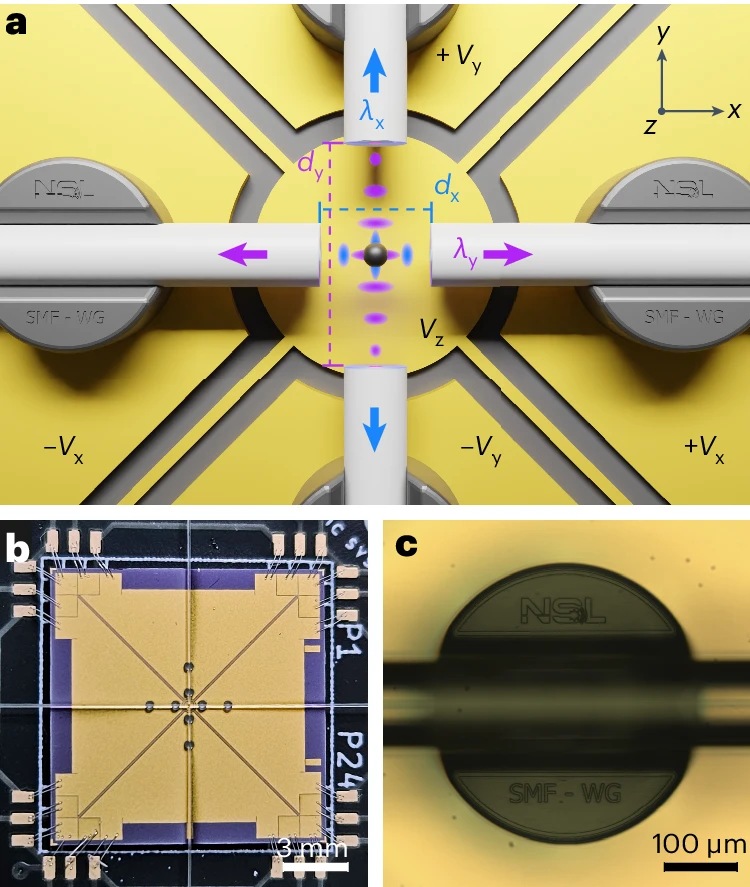
The work of the ETH Zurich researchers offers a glimpse into a future where miniature and integrated levitation systems enable new experimental protocols and applications.
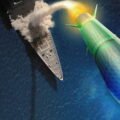
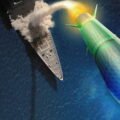
One of the most promising applications is in quantum mechanics. Precise control over the motion of nanoparticles can facilitate the preparation and readout of the complex state, which is essential for quantum computing.
Integrating photonics and nanophotonics with engineered electrical potentials increases control over particle motion, paving the way for scalable quantum systems.
Furthermore, the ETH Zurich team’s approach may influence advances in sensing technologies. By achieving high vacuum levitation, researchers can create more sensitive force and torque sensors, crucial in scientific experiments that require precise measurements at the microscopic scale.
Despite the promising results, some challenges still need to be addressed. The stability and robustness of the levitation system in different environments, the scalability of the technology, and the integration with other quantum systems are areas for future investigation.
The ETH Zurich team is already planning to further improve their platform. Future studies will focus on increasing the detection sensitivity using refractive microlenses and integrating more sophisticated optical elements such as fiber cavities. These advances aim to achieve even greater control over particle motion and pave the way for complex state preparation and readout.
Ultimately, ETH Zurich’s breakthrough in high-vacuum levitation of silica nanoparticles on a chip marks an important milestone in nanotechnology. Its potential applications in quantum computing, sensing technologies and materials science underscore the importance of continued research and development in this area. As technology evolves, it promises to open new horizons for scientific exploration and practical innovations.
“We envision our platform as a stepping stone towards the use of hybrid potentials for quantum experiments based on levitated particles,“ the researchers concluded.
Tim McMillan is a retired law enforcement executive, investigative reporter and co-founder of The Debrief. His writing typically focuses on defense, national security, the Intelligence Community, and psychology-related topics. You can follow Tim on Twitter: @LtTimMcMillan. Tim can be reached by email: tim@thedebrief.org or via encrypted email: LtTimMcMillan@protonmail.com