A collage of five of the types of comb jelly studied. Red coloration as seen in the two specimens on the right is common among deep sea animals. Credit: 2021 Jacob Winnikoff
The bottom of the ocean is not welcoming. there is no light; the temperature is too cold; and the pressure of all the water up there will literally crush you. Animals living at this depth have developed biophysical adaptations that allow them to survive in these harsh conditions. What are these adaptations and how did they develop?
Assistant Professor of Chemistry and Biochemistry at the University of California San Diego, Itay Budin, joined researchers from around the country to study the cell membranes of ctenophores (“comb gels”) and discovered that they have unique lipid structures that allow them to live under intense pressure. Their work appears in science.
Adaptation to the environment
First things first: although comb jellies look like jellyfish, they are not closely related. Comb jellies include the group Ctenophora (pronounced tee-no-for-a). They are predators that can grow as big as a volleyball and live in oceans all over the world and at various depths, from the surface to the deep sea.
Cell membranes are thin sheets of lipids and proteins that must maintain certain properties for cells to function properly. While it has been known for decades that some organisms have adapted their lipids to maintain fluidity in extreme cold—called a homeoviscous adaptation—it was not known how organisms living in the deep sea adapted to extreme pressure, nor whether the adaptation to pressure was same as cold adaptation.
Budin had studied homeoviscous adaptation in E. coli bacteria, but when Steven Haddock, senior scientist at the Monterey Bay Aquarium Research Institute (MBARI), asked if ctenophores had the same homeoviscous adaptation to compensate for extreme pressure, Budin was intrigued.
Complex organisms have different types of lipids. Humans have thousands of them: the heart has different ones than the lungs, which are different than the ones in the skin, etc. They also have different shapes; some are cylindrical and some are cone shaped.
To answer whether ctenophores adapted to cold and pressure through the same mechanism, the team had to control for the temperature variable. Jacob Winnikoff, lead author of the study who worked at both MBARI and UC San Diego, analyzed ctenophores collected from across the Northern Hemisphere, including those living on the California ocean floor (cold, high pressure) and those from the surface of the Arctic Ocean (cold, not high pressure).
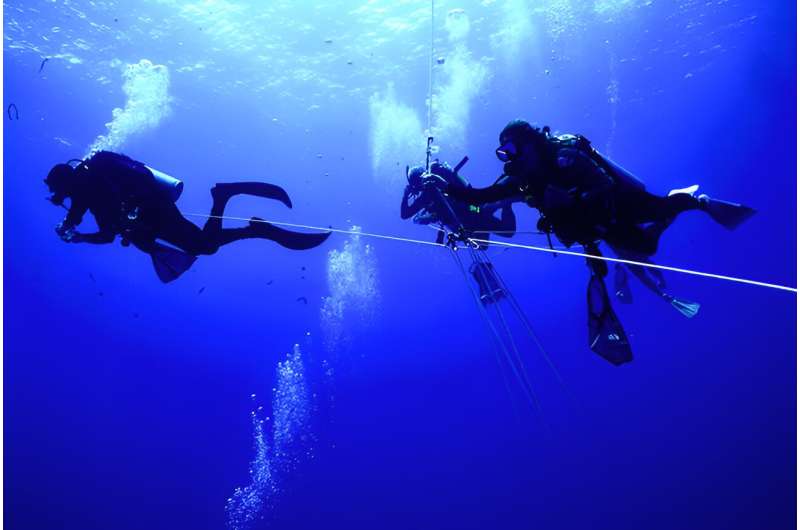
SCUBA diving for shallow water scallops on the Big Island of Hawaii. Most comb jellies live in the open ocean, where divers must use tethers to avoid drifting away. Credit: 2021 Jacob Winnikoff
“It turns out that ctenophores have evolved unique lipid structures to compensate for intense pressure that are distinct from those that compensate for extreme cold,” Budin said, “so much so that pressure is actually what holds their cell membranes together. “
Researchers call this adaptation “homeocurvature” because the shape of the curve-forming lipids is adapted to the unique habitat of ctenophores. In the deep sea, cone-shaped lipids have evolved into exaggerated cone shapes. Ocean pressure counteracts the exaggeration, so the shape of the lipids is normal, but only at these extreme pressures. When deep-sea ctenophores rise to the surface, the exaggerated cone shape reverses, the membranes separate and the animals disintegrate.
Molecules with an exaggerated cone shape are a type of phospholipid called plasmalogen. Plasmalogens are abundant in the human brain, and reduced abundance often accompanies reduced brain function and even neurodegenerative diseases such as Alzheimer’s. This makes them very interesting to scientists and medical researchers.
“One of the reasons we chose to study ctenophores is because their lipid metabolism is similar to humans,” Budin said. “And while I wasn’t surprised to find plasmalogens, I was shocked to see that they make up as much as three-quarters of the lipid count of a deep-sea ctenophore.”
To further test this discovery, the team turned to E. coli, performing two experiments in high-pressure chambers: one with unmodified bacteria and a second with bacteria that had been bioengineered to synthesize plasmalogens. While the unmodified E. coli died, the plasmalogen-containing E. coli strain thrived.
These experiments were conducted over several years and with collaborators in many institutions and disciplines. At UC San Diego, in addition to Budin, whose group performed the biophysics and microbiology experiments, the laboratory of Distinguished Professor of Chemistry and Biochemistry Edward Dennis performed lipid analyzes by mass spectrometry. Marine biologists at MBARI collected ctenophores to study, while physicists at the University of Delaware performed computer simulations to validate membrane behaviors at different pressures.
Budin, who is interested in studying how cells regulate lipid production, hopes this discovery will lead to further investigations into the role plasmalogens play in brain health and disease.
“I think the research shows that plasmalogens have really unique biophysical properties,” he said. “So now the question is, how are those properties important to the function of our cells? I think that’s an important message.”
More information:
Jacob R. Winnikoff et al, Homeocurvature adaptation of phospholipids to pressure in deep-sea invertebrates, science (2024). DOI: 10.1126/science.adm7607
Provided by University of California – San Diego
citation: Under Pressure: How Comb Jellies Adapted to Life at the Bottom of the Ocean (2024, June 27) Retrieved June 28, 2024 from https://phys.org/news/2024-06-pressure-jellies-life-bottom- ocean.html
This document is subject to copyright. Except for any fair agreement for study or private research purposes, no part may be reproduced without written permission. The content is provided for informational purposes only.